Humans are gatherers. Our bank accounts, refrigerators, and attics reflect our desire to store things for future use. This desire holds true with energy. Unfortunately, storing energy is not as simple as putting milk in the fridge. In this post I’ll discuss an emerging form of energy storage that utilizes the marine environment, or marine energy storage.
Intermittency is the kryptonite of renewables; they simply can’t produce power all the time. When there is a spike in the demand for electricity special power plants, usually gas turbine plants, are brought online to help the grid cope. But these peaker plants are very expensive and spend much of their time idle. What’s the solution? Store the excess energy produced during periods of low demand for periods of high demand. This is energy storage.
Grid level energy storage devices convert and store large amounts of electrical energy for later use. They are generally on the megawatt scale and serve unique purposes in support of the grid; like peak shaving or frequency regulation. To be clear: I’m not talking about dinky AA Duracells, this is the big league for energy storage.
Pumped hydro storage (PHS) is the most well known form of energy storage. It’s a simple concept: water is pumped from a low reservoir into a higher one. When electricity demand is high the water is allowed to flow back down to the lower reservoir by way of a turbine that drives a generator. This type of storage can provide power for hours and is often used for peak shaving. PHS systems are generally located near lakes and rivers that have a natural elevation difference. Of course dynamite can help make more of these ‘natural’ features.
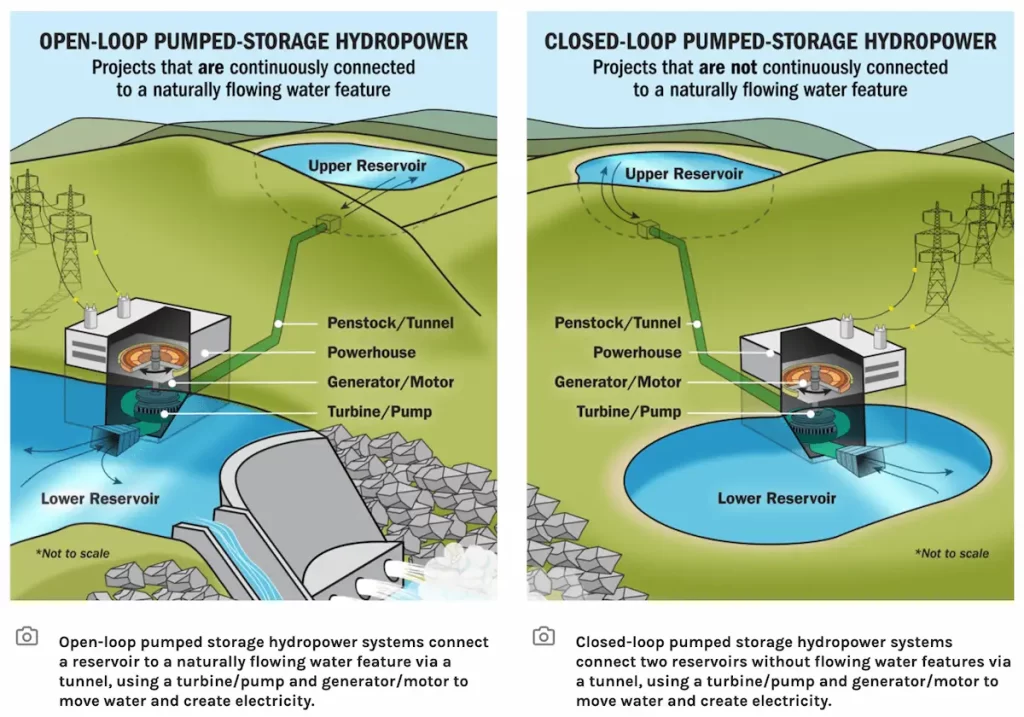
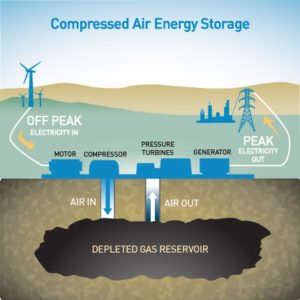
Compressed Air Energy Storage (CAES) is a storage method that squeezes air into a confined space using an air compressor. When extra juice is needed on the grid the compressed air is let out to drive a turbine. A second component to CAES is storing and reusing the thermal energy produced when the air is compressed, which improves the overall efficiency. Underground mines, caverns, or high-pressure tanks are all viable storage areas, but these require unique geological features.
Most marine energy storage (MES) devices are related to their shore-based CAES and PHS cousins. But before we discuss MES we need to understand two key principles: buoyancy and pressure. If you have ever swum underwater you have probably felt the crushing sensation on your ears as you try to dive deeper. You have also probably felt a mysterious force pulling you back towards the surface. This is not an underwater reversal of gravity. These effects are due to pressure and buoyancy.
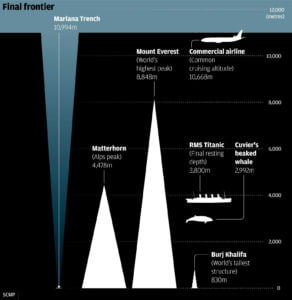
The unpleasant pressure in your ears is due to the weight of the water column above you. It is expressed in force per unit area, like pounds per square inch (psi). At sea level the ambient pressure is a relatively constant 14.7 psi. This is due to the roughly 20 miles of atmosphere above you.
Now pretend you take a sub down to the bottom of the Mariana Trench to Challenger Deep like James Cameron. At 6.8 miles underwater the pressure is an astounding 16,000 psi – more than one thousand times as much as at the surface. The pressure at this depth is roughly equivalent to two Ford F250’s balancing on an area the size of a penny.
What floats? A Monty Python fan might respond with very small rocks or even a witch. Witches aside, in reality what actually determines whether objects float is their buoyancy force. When an object is immersed in a fluid there is a pressure differential between the top and bottom of the object. The bottom of the submerged object feels more pressure than the top resulting in a net upwards force that we call buoyancy.
The buoyancy force equals the weight (different from mass) of the volume of water displaced by the object. This is Archimedes Principle. If the weight of the object is greater than the buoyancy force, the object sinks. If the weight of the object is less than the buoyancy force, it floats. Put another way: if an object’s density is greater than the fluid in which it is immersed, it will sink. This is why it’s difficult to swim down when your lungs are full of air. This is why most oils float on top of water. For my fellow millennials: this is why your iPhone sinks to the bottom of the toilet bowl.
So what does dropping an iPhone in the toilet have to do with marine energy storage? Great question. I’m getting there.
Most marine energy storage devices use very similar principles as PHS or CAES, just underwater. In underwater compressed air energy storage (UWCAES) air is stored in pliable bags on the seafloor. The depth of the water provides the needed pressure to compress the air. When power is needed a valve is opened and the air is allowed to flow out of the bag and to a turbine generator. To charge the system, air is pumped back into the bag.
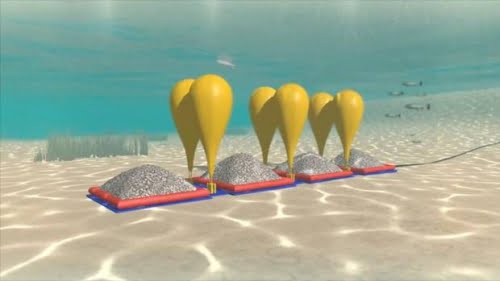
In regular CAES air is stored in a reservoir of a fixed volume. As the compressed air is released the pressure inside the vessel will decrease, reducing the flow to the turbine. Underwater, the pressure acting on the bag remains pretty much constant providing a steady flow of air to the turbine and boosting efficiency.
In underwater pumped hydro storage (UWPHS) seawater is used as the working fluid instead of air. Like UWCAES these devices operate best deep underwater. Unlike UWCAES these devices use rigid spheres of steel or concrete. To discharge, the system allows high-pressure seawater to enter through an opening in the sphere by way of a turbine connected to a generator. To charge the system, the seawater inside the sphere is pumped out.
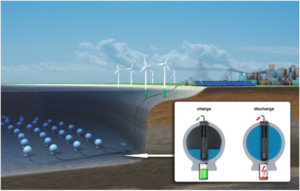
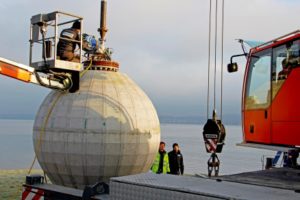
So what’s good about these systems? Marine energy storage can be paired with offshore wind or wave energy conversion devices to make the overall system more efficient. For example a storage device could be integrated into the support or mooring structure of an offshore wind turbine, allowing the combined system to send power to the grid almost continuously. Large-scale systems work well for peak shaving: reducing the load on power generators at peak demand. They are also out of sight for the most part, not impacting the seascape or views.
Some marine energy storage pilot projects have shown round-trip efficiencies on the order of 70-85%, which is commensurate with regular PHS and CAES. Additionally, some developers are claiming that the economics for MES systems are actually quite decent at the commercial scale: around $1,500 to $2,500 per kilowatt. This is on par with high end PHS. However, there are no full-scale systems on the grid yet.
CAES and PHS require specific geographic features. The same holds true for marine energy storage, but there are many more potential sites available. Economically speaking, these systems make the most sense in areas that have relatively deep water close to the coast. Hawaii and California would serve as great sites for example.
The challenges? Well, remember why iPhones sink in toilets? Keeping a large tank or bag that is filled with air on the ocean floor takes a tremendous amount of ballast, or deadweight. Next time you go to the pool take a balloon with you and see how much force it takes to keep it underwater while it’s inflated.
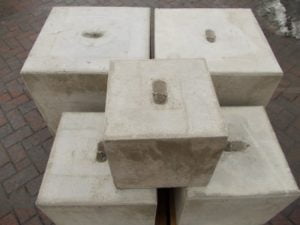
The simplest solution is to use concrete, and lots of it. And then some more for good measure. One MES company suggested that a commercial scale project might use a 40-meter diameter inflatable storage bag. Rough calculations indicate that this bag would need at a minimum 14,000 cubic meters of concrete to offset the buoyancy force of the bag. The article suggests 130,000 cubic meters would be needed. This value might be explained by additional equipment, a high safety factor, and additional holding power required for rough weather.
These devices need high pressure to work best. Shoreside CAES plants typically deliver air to turbines at 650-1090 psi. To achieve this same pressure a marine energy storage device will need to be between 1,475 to 2,460 feet underwater. If anything goes wrong at this depth you’re looking at a costly repair using remotely operated vehicles (ROVs).
There are a few names to keep on your radar for players in this space. Hydrostor, a Canadian firm, has a pilot project in Lake Ontario rated at approximately 1 MW. There is the Stored Energy in the Sea (StEnSEA) project that is being supported by a consortium of German companies, including the Fraunhofer Institute, which just recently completed a small-scale pilot project test as of March 2017. The results look promising, and investors have taken notice.
Marine energy storage is still technologically immature. However, the basic principles behind the designs are tried and true. There are already several pilot projects in the water and I’m sure we’ll see some interesting results and design improvements over the next couple years. Keep an eye to the water, or rather underwater, for the next big development in energy storage.
References:
- Clean Technia
- EIA
- EnergyStorage.org
- Energy Storage Sense
- Lazard Leveled Cost of Storage 2015 Report
- Energy Storage Association;
- Pimm, Andrew; Garvey, Seamus. “Underwater Compressed Air Energy Storage” Chapter 7
- Slocum, A. H. et al. 2013. “Ocean Renewable Energy Storage (ORES) System: Analysis of an Undersea Energy Storage Concept.” Proceedings of the IEEE 101(4): 906–924.